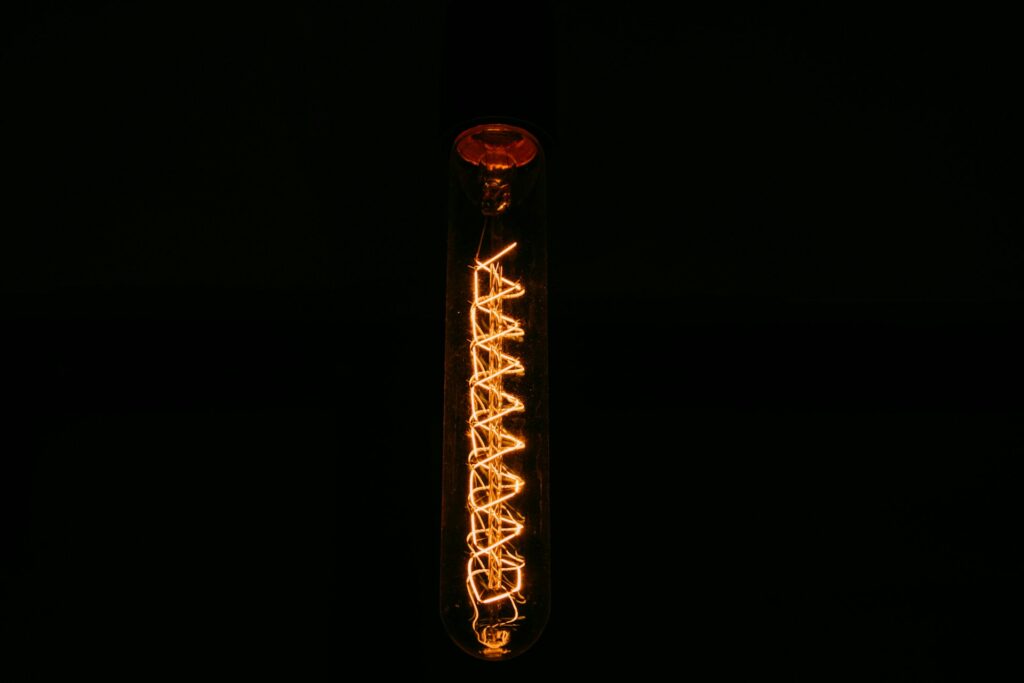
The human genome encodes human life as we know it. Its DNA sequences encode almost 20000 proteins that affect what we look like, how we behave, and how well our bodies operate. Yet in the process of characterizing the human genome, scientists have also uncovered a plethora of other elements. Although these regions in the human genome do not encode a functional protein, they affect how genes are expressed and impact disease severity and outcomes.
That’s not to mention how DNA is structured.
Too often we reduce DNA to strings of four letters: A, T, G, and C. Yet behind these letters are chemical compounds that are bound by specific proteins and generate 3D shapes. In this geometric space, we can learn how our DNA sequences affect who we are. We may also find new ways to treat disease in these structures.
That’s why I’m delighted to interview the new CEO of Enhanc3D Genomics, Hazel Jones. Her previous experience with business planning and operations at AstraZeneca leaves her well-suited to continue pushing the company at the forefront of studying the 3D structures of the human genome.
Now read on to learn more about the intricacies of genome structure and how harnessing it can help scientists treat disease.
The Interview
PN: When we sequenced the human genome, we thought that we could cure all diseases by that point. But even after that, we still have a long way to go. What information are we still missing?
HJ: When the first sequence of the human genome was released in 2003, we believed we could identify the genes that caused every disease we knew. But since then, we’ve come to realise that the race to sequence the human genome was not a race to the finishing line; it was a race to the starting line. Only 2% of the human genome encodes a functional protein. In the other 98%, we encounter gene regulatory elements including so-called enhancers that control which genes are expressed where and when in the human body, and their magnitude. These elements do not encode a protein but rather act as ‘molecular switches’ that control where and when protein-coding genes are expressed. From a disease perspective, enhancers contain most disease-associated gene variants, determined through genome-wide association studies (GWAS).
PN: Tell us a bit more about these disease variants. How do they have such a big role in disease?
HJ: I think it would be best to discuss a specific example: the Sonic Hedgehog (Shh) gene in mice. For video game enthusiasts, the gene is named after the SEGA character Sonic the Hedgehog. In developing limbs, an enhancer sequence located almost a million base pairs away ‘jumps over’ its neighbouring genes to contact the Shh gene and activate its expression. When this enhancer was deleted in a mouse model, the mice are born without limbs. Fascinatingly, single nucleotide changes (i.e. variants) in the human Shh limb bud enhancer cause limb malformations known as polydactyly, as a consequence of altered Shh expression. This is a key example of a disease-associated long-range enhancer with evolutionarily conserved function. Identifying the target genes of these long-range enhancers is impossible without profiling the folding of the genome in 3D.
PN: I can’t imagine this being the only example of 3D genome structures affecting disease onset.
HJ: I wholeheartedly agree. In fact, there are numerous examples of mutations in enhancers and enhancer dysregulation that cause of human disease – and this class of ‘enhanceropathies’ is constantly growing. We are probably still only scratching the surface at the moment, and we aren’t close to unpacking all of these complex relationships. The challenge in linking changes in 3D structure to various disease states lies in determining which target gene a specific non-coding disease-associated region regulates, because like the first example I gave, the majority of enhancers regulate genes further away than the nearest gene via 3D-genome folding events, and thus cannot be predicted without performing a physical 3D-genome mapping exercise.
PN: I believe that’s where your GenLink3DTM platform comes in.
HJ: Yes, that’s right. Our platform uses what’s called Hi-C sequencing to profile a DNA’s 3D structure at a whole-genome scale. It is through 3D structures that a 2 metre long DNA-structure fits within the nuclei of our cells. . Hi-C sequencing fixes DNA-folding loops and then determines which two distant DNA-regions are brought into close physical proximity.
Our GenLink3D pipeline, called “promoter-capture Hi-C (PCHi-C)” however, distinguishes itself by providing 3D-data across all genes in the human genome and their enhancers in a single experiment. This gives us the unique ability to unlock the therapeutic potential of non-coding variants and their target genes.
PN: It’s amazing how we’re now learning about the ways the DNA structure affects who we are. What new insights have you gleaned with the GenLink3D platform?
HJ: Our platform plays a new important role in preclinical drug discovery. More specifically, we revealed that different cell types possess unique 3D genome shapes that fundamentally drive their core identity and function. In a ground-breaking 2016 study we mapped the genome-wide 3D contacts across 17 different cell types of the immune system, creating the first comprehensive immune cell 3D genome atlas.
This atlas then allowed us to finally understand which genes are the long-range targets for 100s of previously known GWAS “risk variants” in ulcerative colitis (UC), opening new doors to the diagnosis and treatment of this condition. Moreover, target and enhancer gene interactions in activated memory T cells, which mediate adaptive immune responses in our bodies, were found to be particularly important in causing their overactivity, which is key to causing gut inflammation.
Interestingly, different cell-types and mechanisms seem to be at play in different patient populations. Our work has also helped to identify a specific single nucleotide polymorphism (SNP) among East Asians associated with genes expressed in monocytes, CD4+ cells, erythroblasts, and B cells, highlighting the power of our technology to pave the way for treatments that are tailored for specific ethnicities or individuals – the key aim of personalised medicine.
Now, we’re looking into obtaining 3D genome folding information from as many human cell types as possible. This effort will give us a complete 3D human genome atlas that we can use to interrogate any gene function in any human cell type.
PN: I can already begin to see the power behind your GenLink3D platform, especially given how high cell and DNA inputs are required in typical Hi-C sequencing protocols.
HJ: Exactly. We have put a lot of effort into developing an advanced version of PCHi-C that allows us to profile rare cell types. Most Hi-C and capture Hi-C protocols require millions of cells to start with; we can now go down to about 50 thousand cells. This substantially increases the range and sensitivity of our sequencing pipelines. Both, in turn, reduce the costs of generating and analyzing the data through bioinformatics.
PN: So now that you’re the new CEO of Enhanc3D Genomics, where do you see your company heading? How would you like to expand your GenLink3D platform?
HJ: So we’re currently in a growth-transition phase. We’re moving away from being a small biotech platform company towards applying our knowledge of 3D genomics to important biological questions and unmet medical needs. To that end, we have identified three main areas:
- Disease knowledge: We believe that understanding disease pathophysiology at the molecular and genetic level is essential to treating diseases. Given that the vast majority of non-coding human genome has yet to be “de-orphaned” for which disease variants regulate which target genes, in which cell-type, our 3D platform has the abilityto uncover hundreds of new disease causation mechanisms for therapeutic exploitation..
- Target identification: Here, we will define which enhancer-target interactions represent effective nodes for novel therapeutic development, by performing key “target-validation” experiments using functional genome editing “knock-out” studies.
- Biomarker prioritization: Through these functional experiments, we can also define the strongest biomarkers for disease onset, improvement and worsening. With this model, we can therefore also impact the field of diagnostics, as well as accelerate drug discovery efforts, thus facilitating true personalised patient care.
- Technology development: we are constantly developing and advancing our cutting-edge technology platforms to remain at the forefront of 3D genomics. Currently, our efforts in this space are focussed on single-cell and long-read sequencing applications, which will expand the range of tissue cell-types that can be interrogated.
To build upon these four areas, we are establishing collaborations with various biopharmaceutical companies across the UK, Europe, and the globe. All of them are dedicated to harnessing the 3D genomics data we provide to identify clearer endpoints in clinical trials and ultimately tailor treatments to patients. We are convinced that a deeper understanding of 3D genomics will catalyse step changes in precision medicine.
Author
-
Paul Naphtali is a seasoned online marketing consultant. He brings to the table three years of online marketing and copywriting experience within the life sciences industry. His MSc and PhD experience also provides him with the acumen to understand complex literature and translate it to any audience. This way, he can fulfill his passion for sharing the beauty of biomedical research and inspiring action from his readers.
View all posts