Disinfectants: an underrated opportunity for environmental sustainability
The discussions about and concerns regarding proper care for our environment have largely focused on reducing carbon emissions. Experts and social media alike have frequently implicated coal, natural gas, and oil as the primary drivers of carbon emissions worldwide. These non-renewable resources are commonly used as energy sources for our cars, homes, and industries. However, the products generated from these resources have been used for another important product: disinfectants.
What is a disinfectant, and what are its ingredients?
A disinfectant is a compound, typically in liquid form, that is used to kill microorganisms and viruses dwelling on a surface. This surface could be a metal table at a dentist’s office, a residential dinner table, or even your hands. There are many kinds of disinfectants based on their chemical composition. Three of these commonly encountered disinfectants are quaternary ammonium compound (QACs)-based disinfectants, chlorine-based disinfectants, and alcohol-based disinfectants (Figure 1). QACs are molecules comprised of a nitrogen atom connected to three other molecules (most commonly an alkyl or an aryl chain) known as R-side groups (Figure 1, top left). QACs can kill microbes by destroying the protein machinery needed to generate energy and by puncturing their cell walls or membranes. QAC-based disinfectants are widely used because of their potent ability to kill all kinds of microbes indiscriminately. Most commonly, QACs are key ingredients in Lysol wipes and disinfectant sprays.
Chlorine-based disinfectants use sodium hypochlorite as the key ingredient (Figure 1, top right). Sodium hypochlorite kills microorganisms by destroying proteins after the compound enters the cell. Chlorine-based disinfectants are most commonly encountered in bleach. Finally, alcohol-based disinfectants are primarily comprised either of ethanol or isopropanol. Ethanol and isopropanol also destroy proteins within the cell and ruptures the microorganism’s cell membranes. Their potent and immediate effect makes facilitates their use for hand sanitizers.
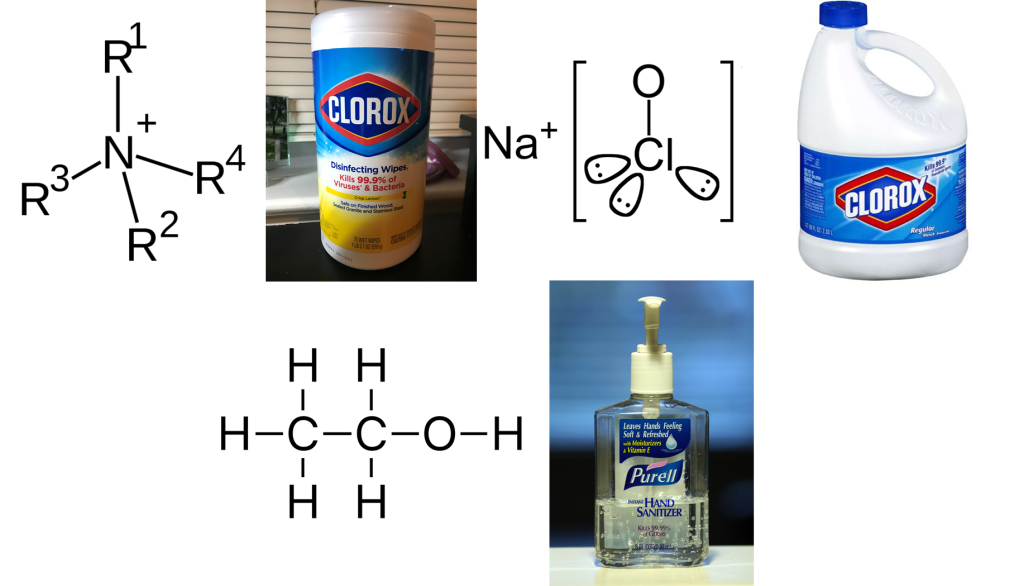
The production and use of disinfectants – how it affects the environment
Since the COVID-19 pandemic began, global disinfectant production and revenues have gone up drastically. In Canada and the UK, revenue from hand sanitizer sales increased from US$17.00m to $29.11m and $69.86m to $111.88m respectively1. Japan’s Kao Corporation, a prominent chemical and cosmetics company, increased hand sanitizer products by 2,000% in April 2020 in response to the acute hand sanitizer shortage when SARS-Cov-2 arrived in Japan2. Clorox also saw a 500% increase in demand for their wipes when the COVID-19 pandemic began and responded by producing 40 million more items in Q1 2020 than it did in the same period the year before3. Lastly, the disinfectant markets are expected to have a compound annual growth rate of 12% in the next 5 years even if the effect is expected to wane as the COVID-19 pandemic progresses4.
The increased use of hand sanitizers when managing the COVID-19 pandemic may coincide with the increased consumption of non-renewable resources and the emission of greenhouse gases. Ethanol-based disinfectants are produced from the dry-milling of corn and sugar cane. However, this process still produces carbon dioxide (CO2), the most prominent greenhouse gas, as a byproduct5. Although the plants being grown for ethanol production can absorb and offset the CO2 being produced, a way to reduce CO2 production would further improve environmental sustainability. Isopropyl alcohol, another common ingredient in hand sanitizers, is produced from propene which is generated from fossil fuel and natural gas production.
The constant wiping down of appliances, desks, and other furniture with Clorox and QAC-based disinfectants can also be toxic to aquatic microorganisms and animals. Wipes that are immediately dumped into the garbage after use will still have QAC-based compounds that are disposed into the environment. The QACs on the wipes could also make their way from sinks and toilet seats to sewage and be dumped into rivers, lakes, and oceans with sewage effluent. In both cases, QACs can seep into aquatic environments at concentrations toxic to fish, algae, and protozoans6. QACs can also interact with chlorine during the wastewater chlorination process to produce N-nitrosamines. The most notorious of these N-nitrosamines is NDMA, which is a known carcinogen. The myriad effects of disinfectant usage on greenhouse gas emissions and ecosystem health mean that a more environmentally friendly disinfectant should be produced.
Bioliquid as a novel disinfectant
In response to the need for a more environmentally-friendly disinfectant, a research lab from Fudan University in Shanghai published a study in PNAS (Proceedings of the Nature Academy of Sciences of the USA) that provides a possible way to produce such a disinfectant. The disinfectant comes in the form of a bioliquid capable of killing 99.99% of bacteria such as Escherichia coli, Staphylococcus aureus, and spores from Bacillus subtilis after 30 minutes of exposure in wet lab experiments7. Electron microscopy images from their study showed that the bacterial cells underwent extensive cell membrane damage after being exposed to the bioliquid. Their bioliquid also inactivated multiple strains of influenza (H1N1, H5N1, H7N9) and did not cause skin rashes in rabbits 24 hours after touching the bioliquid.
The bioliquid that the authors produced was comprised of sawdust, bamboo powder, rice straw, or corn straw. Each of these components was dissolved into ultrapure water and then underwent a process called hydrothermal liquefaction to degrade the powders (Figure 2). In hydrothermal liquefaction, the biomass mixed in water is placed inside a pressurized water environment set at temperatures as high as 270oC. At that temperature and pressure, the biomass solution is broken down into bioliquid. The temperature at which the biomass is exposed ensures that the solids become liquefied without the solution becoming gas at higher temperatures8.
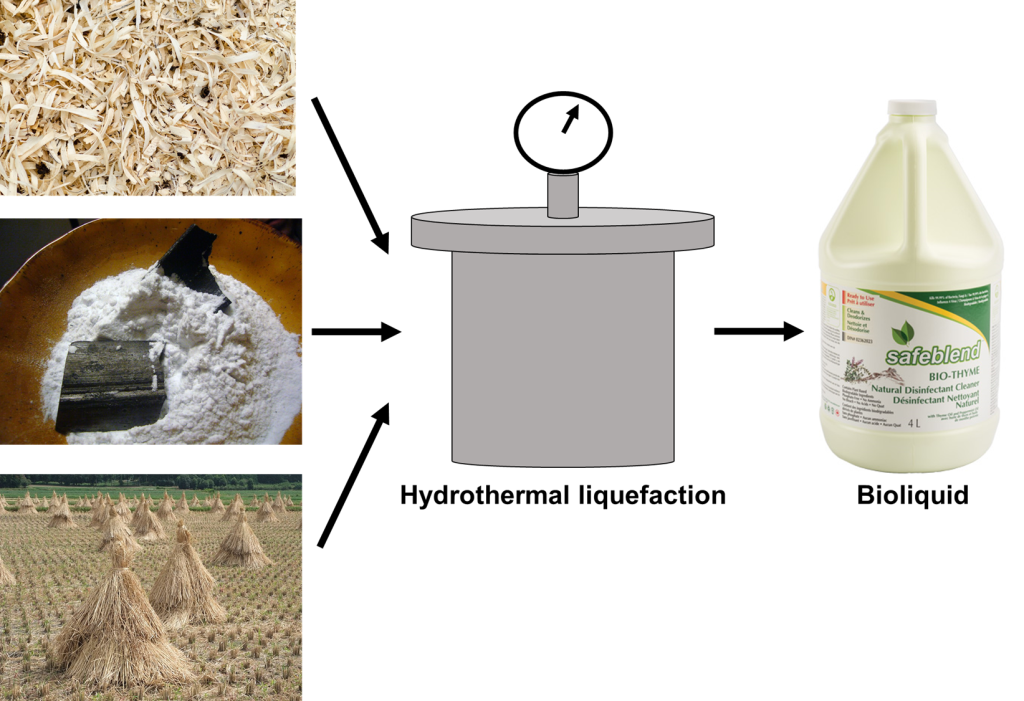
But what was in the heated, pressurized powder that made the bioliquid so effective against all kinds of pathogens? To determine this, the authors used two specialized techniques called mass spectrometry and 3D fluorescence spectroscopy. Both methods are used to learn more about the makeup of the molecules within the bioliquids before and after the hydrothermal liquefaction. The wood components obtained from sawdust, bamboo powder, and straw, are comprised of lignin polymers which the authors observed using spectroscopy (Figure 3). Once the biomass was degraded, the authors identified a series of lower molecular-weight phenol and ketone structures in the bioliquid (Figure 3). Both lighter-weight molecules are capable of killing bacteria, evidenced by the study’s findings.
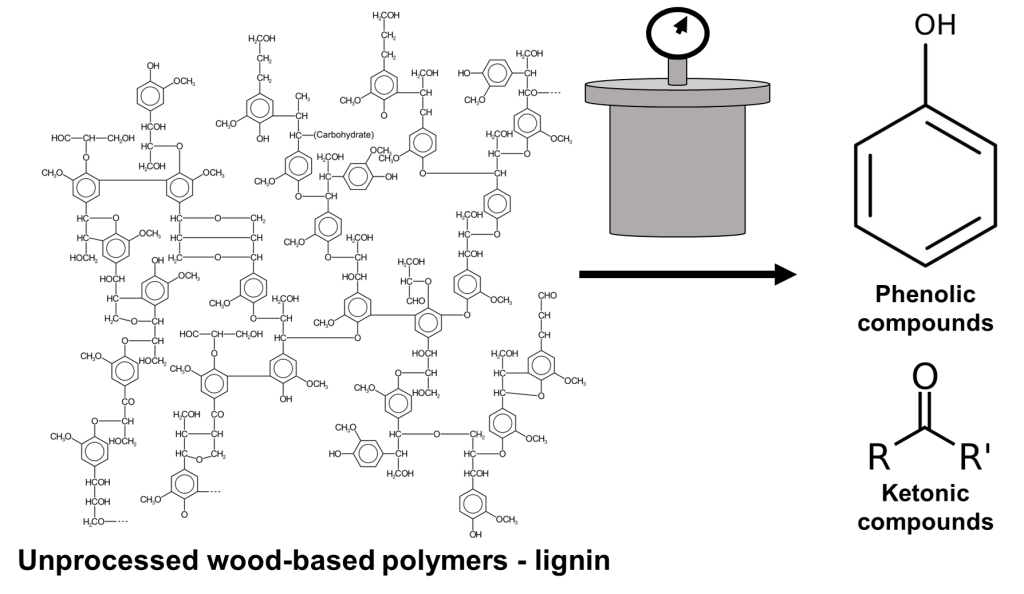
How cost-effective and sustainable is this procedure?
The authors claimed that producing a single kilogram of their disinfectant only costs 4 cents. That’s far lower than the $4 to produce a kilogram of phenolic disinfectant and the 50 cents per kilogram for producing bleach-based disinfectants. However, this value was based on the amount of the bioliquid the Shanghai lab produced with their protocol compared with costs obtained in the literature. The question then arises as to how the bioliquid would be used. Is it to be used as bleach based on the cost comparisons with hypochlorite-based disinfectants? What about hand sanitizers or Clorox? I think more clarification on this point would have been useful for the paper. If the disinfectant was to be used as hand sanitizer, it would have been more prudent to perform the cost comparisons with alcohol-based disinfectants for instance.
As for environmental health, I am a little concerned about phenol-based compounds making their way to the aquatic environment. Phenols are highly toxic to aquatic organisms and humans9. While the rabbit feet were fine after touching the phenols, I think more well-controlled, humane experiments on aquatic organisms and other animal models would have shed more light on this point. Nevertheless, it helps that the resulting products are phenol-based compounds as opposed to phenol itself. The lack of CO2 emissions calculations also left me wanting because of their focus on minimizing humanity’s carbon footprint. I think more questions about environmental sustainability need to be answered before the bioliquid can be called as such with certainty, but the work does move in the right direction.
What’s next?
Bioliquids have primarily been used as a sustainable alternative from oil and natural gas for fuel. The results from this study add a possible application for bioliquids as a more environmentally friendly disinfectant. That being said, much more can be done to make bioliquid-based disinfectants a reality. An evaluation of the bioliquid’s safety with human application beyond a 24-hour assay with rabbit feet and earthworms would better determine whether the bioliquid is safe to use. Second, a means to test the bioliquid on surfaces in a real-world application would help better assess whether the bioliquid works in everyday life. Finally, the bioliquid’s byproducts should be assessed to ensure minimal negative effects on the environment after usage. A lot of work is still left for this bioliquid to become marketable and so current disinfectants are still necessary for keeping our home and work environments clean. Nevertheless, work is underway to develop more environmentally friendly disinfectants, as seen by the study. This work provides promise for maintaining cleanliness while taking care of the environment.
The link to the paper I talked about can be found here: Biosafety of human environments can be supported by effective use of renewable biomass | PNAS
References
- “Hand Sanitizer” Statista.com. 2021. https://www.statista.com/outlook/consumer-markets.
- Kyodo News. April 16th, 2020. https://english.kyodonews.net/news/2020/04/5e47d5018f49-feature-japan-breweries-answer-call-for-strong-alcohol-amid-sanitizer-shortage.html.
- Blayne, A. and Stelloh, T. May 7th, 2020. NBC News. https://www.nbcnews.com/nightly-news/clorox-ramps-production-wipes-disinfectants-fight-against-coronavirus-n1201561.
- Klemeš, J.J., Fan, Y.V., and Jiang, P. (2020). The energy and environmental footprints of COVID-19 fighting measures – PPE, disinfection, supply chains. Energy (Oxf.) 211, 118701.
- US Department of Energy. “Ethanol Production and Distribution”. https://afdc.energy.gov/fuels/ethanol_production.html#:~:text=Most%20ethanol%20in%20the%20United,distillers%20grains%20and%20carbon%20dioxide.
- Hora, P.I., Pati, S.G., McNamara, P.J., and Arnold, W.A. (2020). Increased Use of Quaternary Ammonium Compounds during the SARS-CoV-2 Pandemic and Beyond: Consideration of Environmental Implications. Environ. Sci. Technol. Lett. acs.estlett.0c00437.
- Yu, F., Zhao, W., Qin, T., Zhao, W., Chen, Y., Miao, X., Lin, L., Shang, H., Sui, G., Peng, D., et al. (2022). Biosafety of human environments can be supported by effective use of renewable biomass. Proc. Natl. Acad. Sci. U S A 119, e2106843119.
- Elliott, D.C., Biller, P., Ross, A.B., Schmidt, A.J., and Jones, S.B. (2015). Hydrothermal liquefaction of biomass: developments from batch to continuous process. Bioresour. Technol. 178, 147–156.
- Babich, H., and Davis, D.L. (1981). Phenol: A review of environmental and health risks. Regulatory Toxicology and Pharmacology 1, 90–109.
Author
-
Paul Naphtali is a seasoned online marketing consultant. He brings to the table three years of online marketing and copywriting experience within the life sciences industry. His MSc and PhD experience also provides him with the acumen to understand complex literature and translate it to any audience. This way, he can fulfill his passion for sharing the beauty of biomedical research and inspiring action from his readers.
View all posts